|
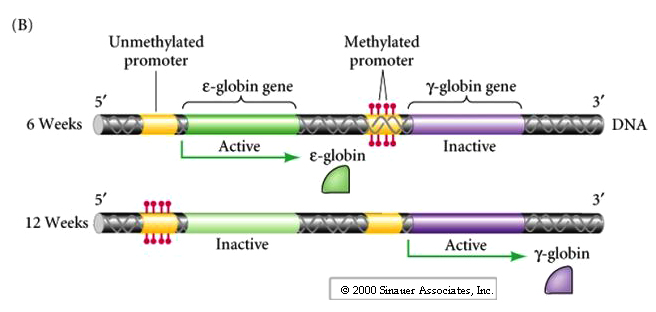
The above figure shows an example of differential gene expression regulated by methylation of the promoter region. This happens to be an example of a gene regulated differentially at 6 weeks and 12 weeks of embryogenesis and not differentially in male and female derived genes.
Why are the male and female gamete nuclei distinct in their ability to support development? Why are they differentially methylated (imprinted) so that different genes are activated or repressed in the sperm and egg DNA?
At least part of the explanation lies in the genetic conflict between male and females. The best strategy for reproductive success may be somewhat different for the male and female. The male wants to maximize the potential survival of embryos carrying his DNA, thus he contributes DNA that is imprinted in a way that will try to maximize the resources taken from the female for "his" embryo. However, the best strategy for the female is to regulate the resources given to the embryo so that she can save enough resources for potential future offspring. She can only have progeny with herself, while the male can have progeny with many females. Thus the female imprints the DNA of her gametes to counteract the "selfishness" of the male derived DNA. Remember, there can be a genetic conflict between the baby and the mom because the baby is only related to mom by 50%.
As you would expect from the above discussion, one class of genes that are differentially regulated by imprinting are those involved in energy metabolism. On example that you should remember is Insulin-like growth factor II (Igf2) and Insulin-like growth factor receptor (Igf2r). Expression of the Igf2 protein leads to an increase in glucose uptake by the embryo (from the mom). This gene is active in sperm derived genes and inactive in female derived genes (through methylation). The Igf2r protein binds to the Igf2 protein causing inactivation and premature degradation. Igf2r is active in female gamete DNA and repressed in the male gamete DNA. When a mouse pup inherits a deletion of Igf2r from the father, the pup develops normally. However, when a pup receives the same mutation from the female the results are disastrous. Now the Igf2 produced by the male DNA causes the placenta to enlarge and glucose uptake from the Mom to increase. The pup dies late in embryogenesis in part because it is grossly enlarged (30%).
There is no way to make sense of these patterns of male and female specific imprinting during gametogenesis unless we invoke the conflict between male and female reproductive strategies and the evolutionary "arms race" for reproductive fitness. We cannot explain the differences between the male and female imprinting as some optimization bioengineering. Thus, be wary of always trying to explain a developmental process or mechanism as a result of "optimal" design! If you are interested in reading more about genetic conflict and imprinting check out this IMPRINTING site and this article by David Haig at http://www.edge.org/3rd_culture/haig/haig_index.html.
Experimental evidence to date suggests that most developmental failure of NT embryos is due to the inability to reprogram the epigenetic profile of the genetic donor nucleus to that of a normal zygotic nucleus. About 60% - 70% of the embryos from somatic nuclear transfer survive to the blastocyst stage. Only 10 20% of the embryos derived from embryonic stem cell nuclear transfer survive to the blastocyst stage. The main difference may be most somatic cells are in G0, while most ES cells(60%) are in S phase of the cell cycle and only a few in G0 . However, ES cell nuclear transfer embryos that reach the blastocyst stage have a 10 20 fold higher probability of development to birth than embryos derived from somatic cell nuclear transfer.
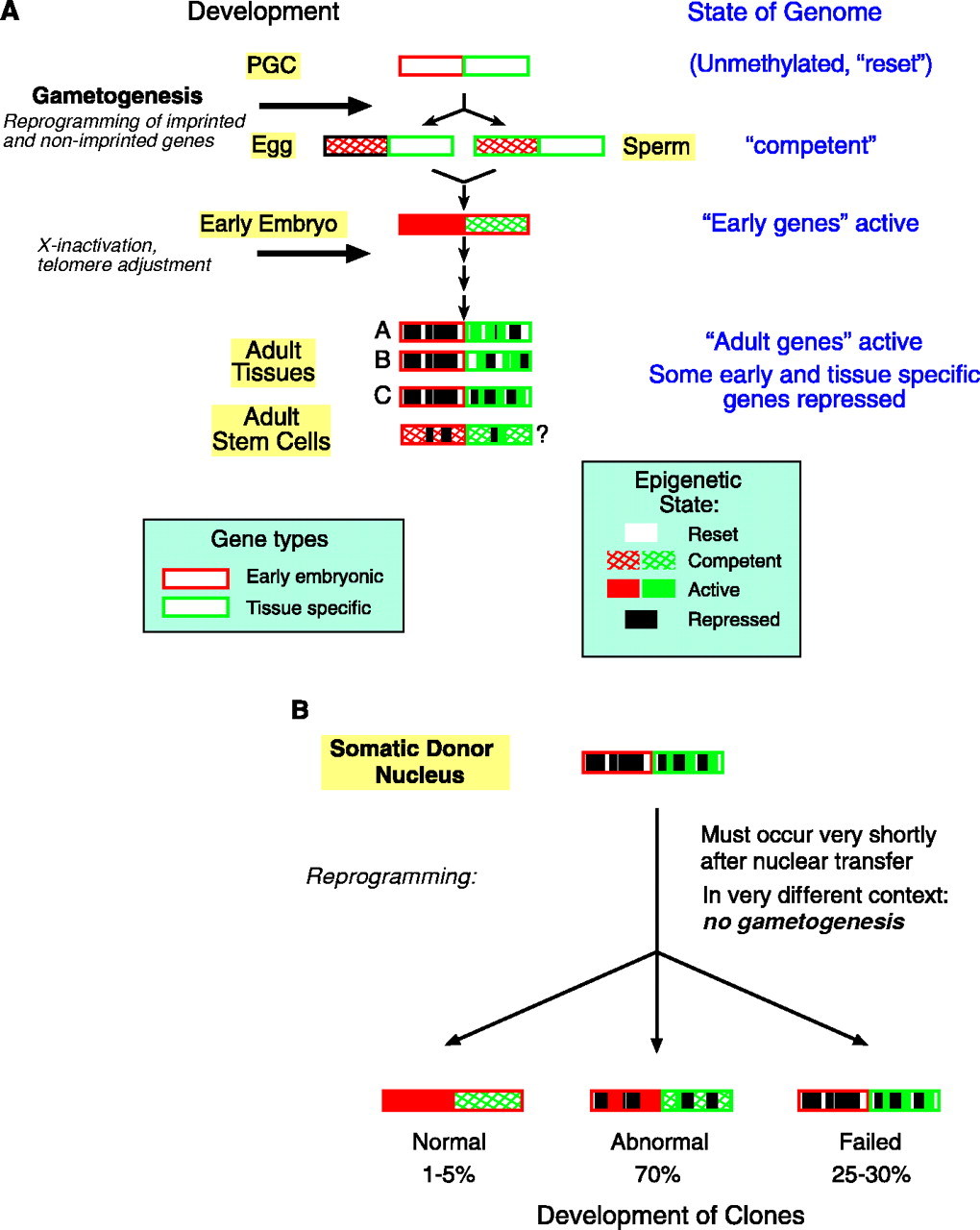
The above figure describes the epigenetic state of the genome as development progresses and explains why cloning by somatic cell nuclear transfer is prone to failure.
Are any clones "normal"? When examined closely most clones derived from somatic cell nuclear transfer show disturbing defects in many organ systems, including the immune and nervous systems. At this time (2012) it would be extremely unethical to try cloning a human via somatic cell nuclear transfer. However, remember that there is nothing new or mysterious about human clones. Maternal twins occur naturally and are genetic clones.
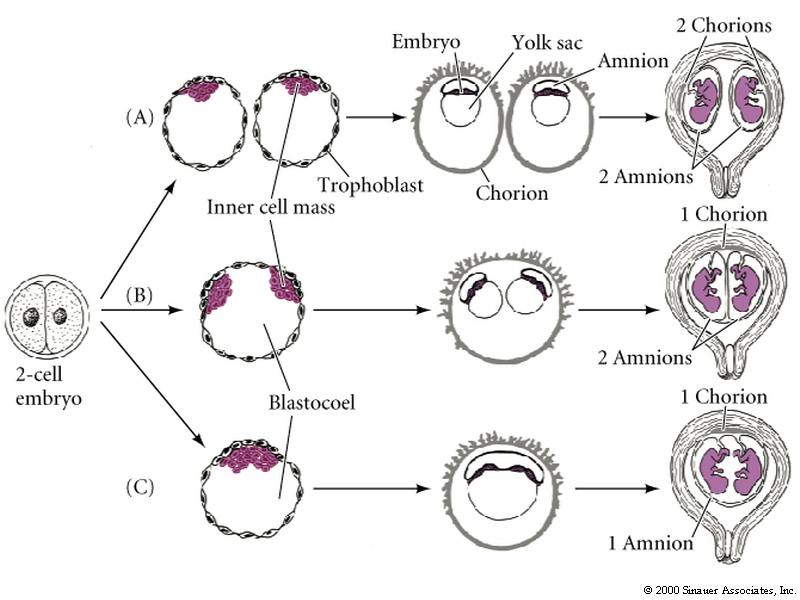
In fact genetic twins illustrates an important process of embryonic development. There is a tremendous potential for regulation of cell number and cell fate early in development. Embryonic Stem (ES) cells are totipotent and can easily adjust their fate to compensate for changes in cell number. This is shown experimentally in the next figure where blastomeres from two normal embryos are fused to form a single embryo. The resulting embryo is perfectly normal in size, but is genetically mosaic showing that blastomeres from the two embryos contributed equally to its development.
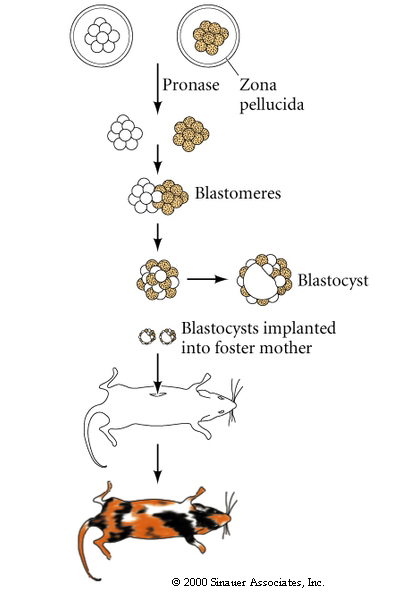
The technique of isolating ES cells, manipulating them in culture and then injecting them back in a developing blastocyst is the basis for many important cloning techniques.
The process of therapeutic cloning shows great promise for the treatment of many human diseases and relies on the totipotent nature of ES cells. The following figures illustrate the process. This interview with Doug Melton illustrates the ethical dilemma a scientist faces.
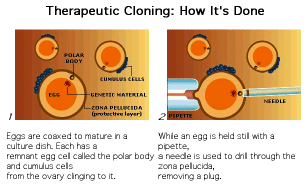
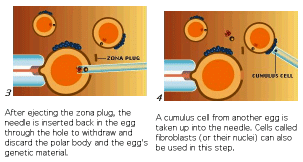
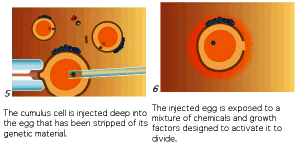
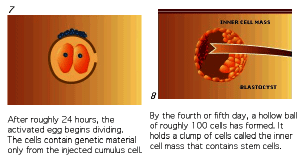
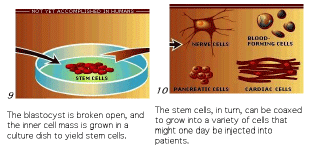
The next figures from your textbook and from the Yamanaka Logic Commentary shows a summary of techniques to create totipotent stem cells.
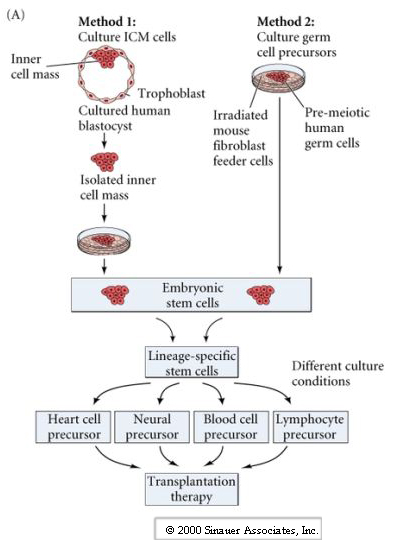 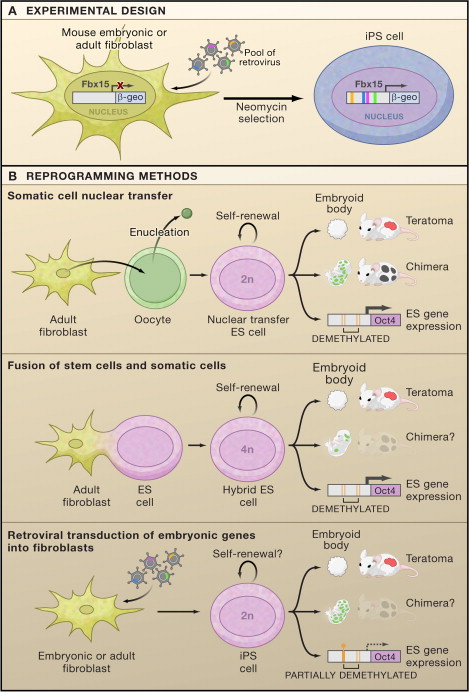
The last set of figures describes the process of gene targeting by homologous recombination and is described in detail in your text and in the handout Mouse Gene Targeting. I cannot over emphasize the importance of this technique. Not only does it allow us to make "gene knockouts" of any mouse gene and generate disease models, but it also makes possible the testing of genetic therapies by making "knockins". It is also obviously the basis for any human engineering of the germ line or of stem cells. Below are the pictures of Mario Capecchi and Martin Evans, both make important contributions to the field of gene targeting by homologous recombination. Martin Smith was instrumental in the identification and characterization of mouse ES cells. Mario Capecchi devised techniques for gene targeting by homologous recombination in mouse ES cells.
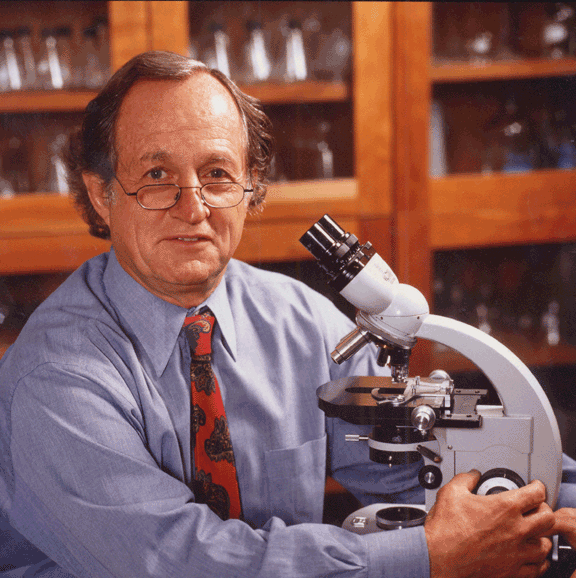 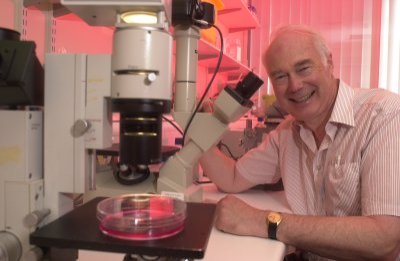
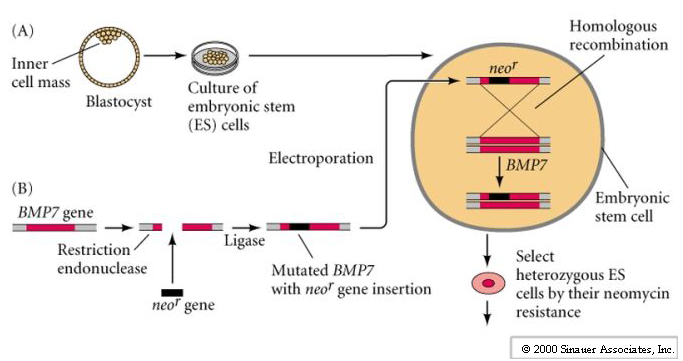
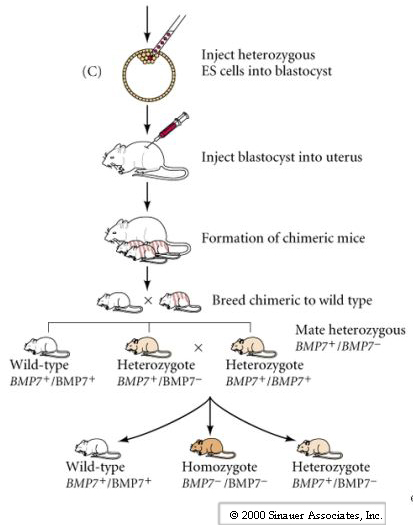
New technique for gene targeting has recently been developed that may supplant gene targeting by homologous recombination (Science Review CRISPR/CAS9). This new targeting technique is so efficient that scientists are beginning to make primate models of human diseases (Nature Focus). Many mouse models for human diseases such as Alzheimers, Autism, Huntingtons, Parkinsons, Schizophenia, and many others do not faithfully phenocopy the human diseases. A more detail description of technique can be read here in this Nature article. The efficiency of this targeting technique may be so good that the individual zygotic nuclei of the single celled embryo may be targeted by cell injection. What type of gene therapy should be allowed? These techniques for making transgenic animals could readily be applied to humans for "personel enhancement". Many scientists are proposing and making human-"animal" chimeras for research purposes (see also this article). Others are proposing to use animal eggs to make human stem cells (CRISPR/CAS9 probably makes this obsolete). Your generation will be faced with deciding these important ethical issues.
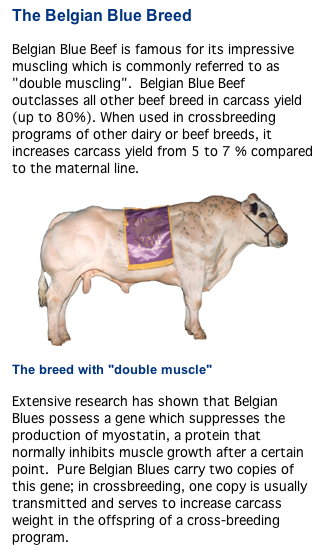
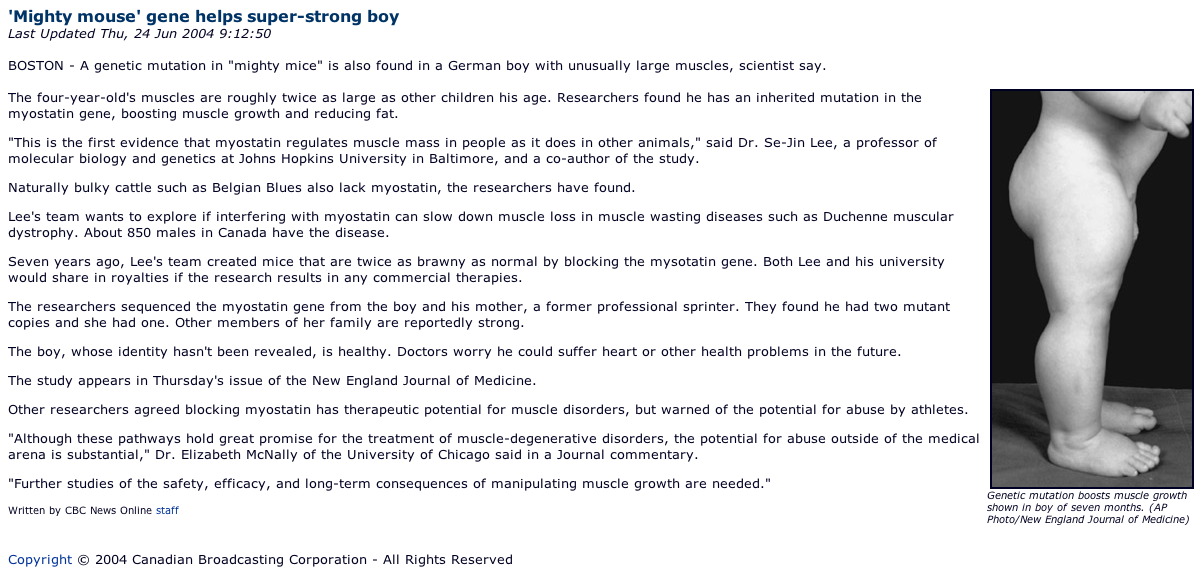
Genome Comparisons
In the last several years the entire genomes of many metazoan organisms have been completely sequenced, including humans. What insights into development have we gained from this vast deluge of sequence information? Perhaps the most intriguing and paradoxical finding has been the discovery that the human genome only contains about 25,000 genes instead of the long predicted 100,000. Furthermore, the comparison of the C.elegans genome of 19,000 genes and the fly genome of 14,000 genes makes the human genome look especially puny! It is obvious from these numbers that the complexity of an animal cannot be linearly accounted for by the number of genes. The worm is by almost any criteria a simpler organism than the fly. The worm only has about 1000 somatic cells total, only 302 neurons. The fly has 100,000 cells or more and much more complex behaviors, eg., Flying! And yet a fly is built with significantly fewer genes than a worm! As discussed in the handouts, objectively comparing the complexity of a human (with 100 trillion cells) to a worm or fly is difficult, but we would all agree humans are at least several orders of magnitude more complex than worms or flies.
The sequence data does reveal some interesting differences between humans and simpler organisms. Humans have evolved a few new classes of protein domains (about 7%), and have expanded numbers of proteins involved in immune and nervous system functions. Other differences that may be important in accounting for the differences in complexity are an increased use of alternative splicing to make more multitask proteins, more complex post translational modifications (carbohydrates), and perhaps most important, larger and more complex regulatory domains. There is still some data that suggests 100,000 or more mRNA transcripts (EST data). But others argue the large number of ESTs is just a technical artifact. The handout on gene number "What if there are only 30,000 human genes?" discusses different ideas of complexity. Until there is some definitive data I tend to favor the idea that our complexity resides in a more complex regulator region. (See Encode Project Publications for latest on "Junk" DNA)
See http://mkweb.bcgsc.ca/circos/
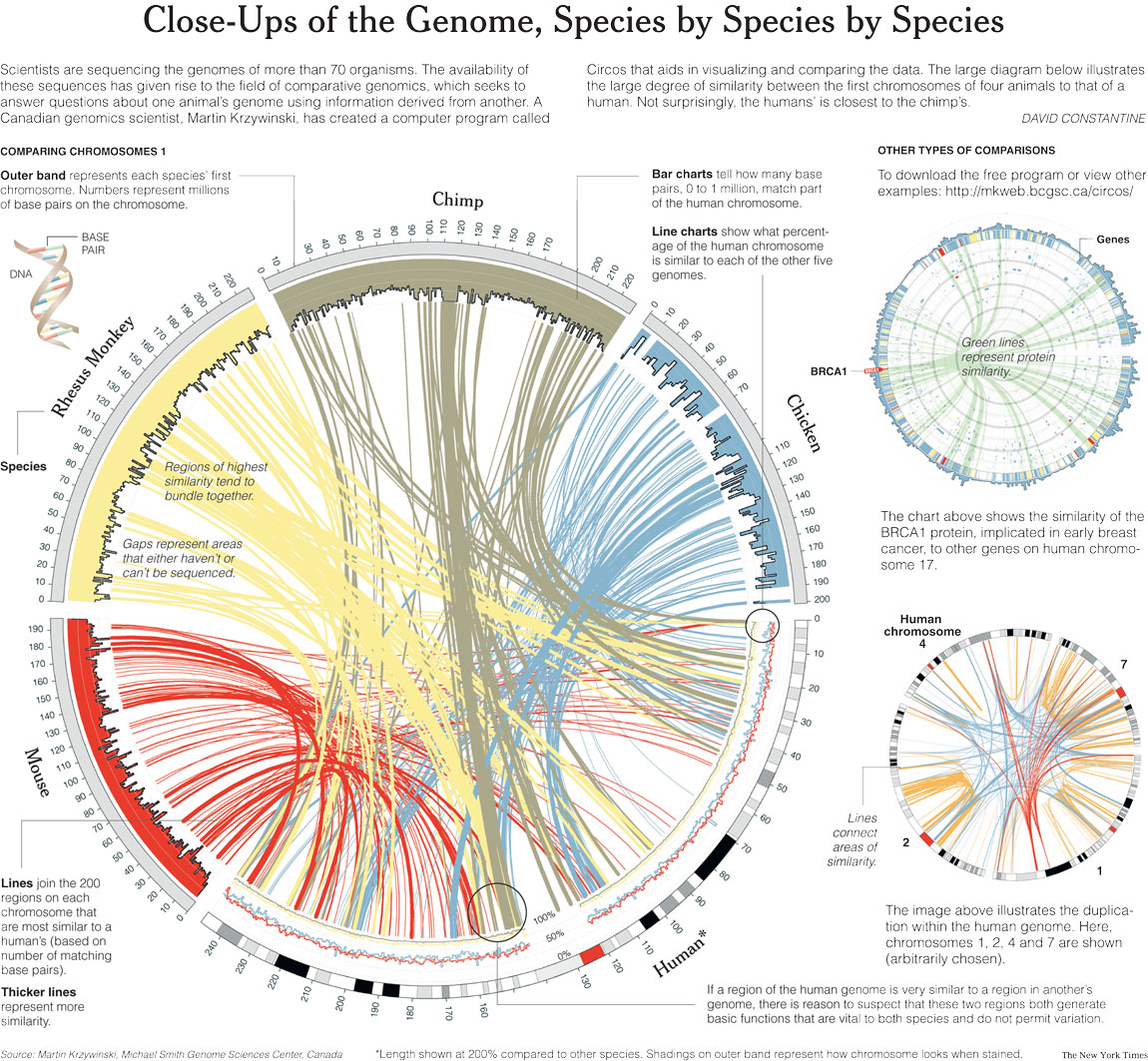
|
|
|